Recipe for a spin-orbital liquid
An international team of scientists has observed an exotic quantum state of matter: a spin- orbital liquid formed on the pyrochlore oxide Pr2Zr2O7. Here, both spin and orbital degrees of freedom remain dynamic down to extremely low temperature. It is known from the long history of condensed matter physics that suppressing orbital order down to low temperatures is extremely difficult, a precondition for the next tricky step of obtaining a spin-orbital liquid state. Pr2Zr2O7 serves as a rare counterexample in which spins and orbitals are interlocked, so that fluctuation of one necessitates fluctuation of the other.
The work of a group assembled by physicists at the University of Tokyo, including scientists from the MPI for the Physics of Complex Systems in Dresden and for Solid State Research in Stuttgart, the Helmhotz Zentrum Dresden-Rossendorf, ICTS-TIFR in Bangalore and Johns Hopkins University in Baltimore, is published in the current issue of Nature Physics.
Introduction
We know that people act differently in a group than when they are alone. Interestingly, this applies to atoms as well. The Nobel-prize winning physicist P. W. Anderson once put “More is different”, which explains unpredictable phenomena in a complex system that cannot be simply understood by the sum of its microscopic constituents. This idea lies at the heart of modern many-body physics, perhaps most famously in the case of superconductivity where pairs of electrons enter a cooperative state which carries electrical currents without resistance – as used in superconducting maglev nowadays.
Recently, macroscopic quantum entanglement which appears in quantum many-body systems, is receiving much attention because the manipulation of such entanglement effect should lead to realization of a series of next-generation technologies, ranging from quantum computing to quantum cryptography. The international research team focused on an exotic many-body state called “spin-orbital liquid” to advance the search for long-range entanglement effects.
Pursuing “liquid” in solids
In nature, matter mainly exists in the forms of solid, liquid, and gas. In solids, all the atoms are periodically arranged and remain immovable from their own sites, while particles in liquid and gas are not that “well-behaved” but move rather randomly. The notion of states of matter can be applied to wider topics as well, such as magnetism. At low temperatures, spins, the most basic building block of magnets, tend to order parallel or antiparallel to each other, forming a neatly aligned “solid” state.
Interestingly, spins are like humans, that they also feel comfortable to behave like everybody else. However, in the past decades, “quantum spin orbital liquid” is proposed as a counterexample to the conventional form of magnetism, in which spins and orbitals refuse to form any patterns and remain “liquid” at low temperatures inside solids.
Such a spin-orbital liquid is hard to realize because spins and orbitals like to go their own way. Spins are driven by low energy spin-exchange interactions, while orbitals are “pulled” by surrounding crystal lattice which has much higher energy scales, into ordered state. In this way, it is considered almost impossible to have high- energy orbitals and low-energy spins fluctuate simultaneously.
Bringing down the energy scale of “orbitals”
The research team proposed a new route to realize spin-orbital liquid in a Praseodymium based mineral, Pr2Zr2O7, also known as a quantum spin ice candidate. Pr ions form a pyrochlore lattice structure, which is a corner-sharing network of tetrahedra, and realizes an oxidation state of Pr3+, which has two 4f electrons. “The fact of possessing even number electrons, which we call ‘non- Kramers’ system, is actually the key to realize this spin-orbital liquid.”, said Dr. Nan Tang from University of Tokyo. The lowest-energy state of Pr2Zr2O7 is a non-Kramers doublet, which can be described by electric quadrupolar moments (orbitals) [Sx, Sy components] as well as a magnetic dipolar moment (spin) [Sz component].
Now the energy scales of orbitals and spins meet! When one of them fluctuates, the other fluctuates as well, which means a spin-orbital liquid can be brought to life on this setting. Unfortunately, as a drawback of all non-Kramers systems, the requirements of crystal quality becomes extremely strict. Since non-Kramers ion Pr3+, unlike its Kramers counterpart (possessing odd number electrons), is not protected by a particular symmetry called “time reversal symmetry”, slight amount of disorder can affect the crystal environment and alter its physical properties. Therefore, a normal high-quality sample used for conventional Kramers system would not be good enough.
This time, the research team succeeded in growing an extremely high-quality Pr2Zr2O7 single crystal. It is obtained by the floating-zone method, in which a polycrystalline rod is melted at temperatures higher than 2000℃ and then the melted
ingredient crystallizes into a single crystal via cooling. The team of Prof. Satoru Nakatsuji came up with the strategy of making the polycrystalline rod much thinner than previous, which, in principle, should result in a more homogenous single crystal. However, such a thin rod is easily broken and requires more precision when being set inside the synthesis apparatus. “We have overcome such technical difficulties, with an optimized recipe and improved synthesis conditions over time, which finally leads to an extremely pure Pr2Zr2O7 single crystal”, said Dr. Kenta Kimura from University of Tokyo. Its top quality is confirmed by both crystallographic analysis from synchrotron X-ray and physical property measurements including magnetization, nuclear quadrupole resonance, and specific heat.
Spin ice physics adds some more flavour
Furthermore, the authors have performed thermal expansion and magnetostriction measurements which measure the length change of samples with respect to temperature and magnetic field, respectively (Figure 4, left), along with ultrasound and dielectric constant measurements. They found no evidence for long- range order (i.e., spins form a static pattern throughout the crystal) of Pr2Zr2O7 down to extremely low temperatures in all the measurements, which, along with other experimental data, points to a dynamic state. To be noted, the length change of insulating materials at low temperature are extremely small and its measurement requires a resolution equivalent to a ping-pong ball on the background of Mount Fuji. Moreover, the generation of extreme low temperature (near -273℃) requires dilution refrigerator which is necessary for the research of quantum phenomena, including quantum computing.
By applying magnetic fields, they observed evident increase at the same magnetic fields in all measurements, which is called metamagnetic anomaly.
Metamagnetic anomaly, a characteristic feature of spin ice, serves as a boundary line to separate a “gas” phase and a “liquid” phase in Pr2Zr2O7. In this sense, the observation of metamagnetic anomaly serves as an example of the manipulation of macroscopic quantum entanglement by magnetic field. The theorists team, Prof. Roderich Moessner from MPI and Prof. Subhro Bhattacharjee from Tata Institute of Fundamental research, also discover that the lattice can actually enhance spin-orbital dynamics and stabilize such a topological quantum spin ice state, since orbitals couple with crystal lattice directly.
Beauty of many-body physics
Pr2Zr2O7 is a quantum material with a complex interplay among spins, orbitals and lattice degrees of freedom. Any of the interactions or electronic correlations become too strong and overwhelm one another, the system would suddenly fall into a state of magnetic, electrical, or structural order. The team's extremely pure Pr2Zr2O7 has a fine balance of various interactions and thus succeeded in observing spin-orbital dynamics.
Quantum many-body phenomena, in which various degrees of freedom are intricately intertwined, are a treasure trove of new discoveries, and their quantum entanglement effects are an attractive research topic for next-generation quantum technologies. “The spin-orbital liquid is an important concept that embodies quantum many-body physics and could turn into a building block for future quantum technology.” said Dr. Nan Tang. “This research is expected to serve as a guideline for the design of materials to realize spin-orbit liquids in the future and provides insights for the manipulation of quantum entanglement.” added Prof. Satoru Nakatsuji.
Wissenschaftlicher Ansprechpartner:
Dr. Nan Tang1,2; Prof. Roderich Moessner3; Prof. Satoru Nakatsuji2 nan.tang@uni-a.de; moessner@pks.mpg.de; satoru@phys.s.u-tokyo.ac.jp
University of Augsburg1; University of Tokyo2; MPI for the Physics of Complex Systems3
Die semantisch ähnlichsten Pressemitteilungen im idw
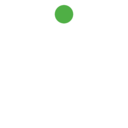