How bacteria actively use passive physics to make biofilms
When we think about bacteria, we may imagine single cells swimming in solution. However, similarly to humans, bacterial cells often socialize, using surfaces to coalesce into complex heterogeneous communities called biofilms. Within a group, bacteria in the biofilm are extremely robust in resisting various environmental stresses – a crucial feature making biofilm-associated infections extremely difficult to treat with antibiotics. For over 50 years, biofilm research has centered around the biological processes which allow biofilms to thrive and become tolerant to antibiotic treatment.
In recent perspective work published in Proceedings of the National Academy of Sciences (PNAS) researchers at the Max-Planck-Zentrum für Physik und Medizin, Erlangen, in collaboration with partners from the Hebrew University of Jerusalem and Harvard Medical School, shared their insights into how bacteria rely on physical processes to form and maintain biofilms, including survival under extreme stress conditions. This work highlights how, in addition to biomolecular details of signaling and regulation, biophysical interactions play an important role in bacterial life cycles and infection.
Biofilms as tissues
“Biofilm research has evolved from the perception of bacteria as single cells (as was originally discovered by Antonie van Leewenhawk) to the realization that given the right conditions bacteria aggregate into groups of cells that make biofilms”, shared Prof. Roberto Kolter (Harvard Medical School, Boston, USA). A recent advance in biofilm research is their comparison with human tissues due to their complexity and heterogeneity. Sometimes, biofilms are even considered to be continuous parts of human tissue, as exemplified by oral biofilms on our teeth or the microbiome residing in human guts. One of the remarkable features of biofilms, shared with eukaryotic tissues, is that bacteria embed themselves in the self-secreted extracellular polymeric matrix which holds the cells together, confers biofilms’ mechanical stability, serves as a reservoir of water and nutrients, and protects against antimicrobial agents. Prof. Liraz Chai (Hebrew University
of Jerusalem, Jerusalem, Israel and Max Planck Queensland Centre, Queensland University of Technology, Brisbane, Australia), suggested: “We encourage zooming out the discussion from extracellular matrix to extracellular space. The extracellular matrix is a single component in a unique milieu, where it is soaked in water and dissolved nutrients, signaling molecules, waste products, and metal ions. Within the milieu, a broad range of physical processes directly affect the biofilm physiology as molecules and bacteria mutually affect each other.”
Where physics unfolds
Water is indispensable for all living organisms and dominates the extracellular space in biofilms. Due to osmotic effects, extracellular matrix can soak up water from the environment and helps biofilms to expand, similarly to a sponge. Evaporation of water from biofilms’ surfaces can drive the flows of water bringing fresh nutrients, when water evaporates from plants’ leaves. Sometimes biofilms even form vasculature-like channels, enabling the flow of water to where it is most needed. When water becomes scarce, biofilms might invoke desiccation stress responses by retaining water in the extracellular space. They can even turn their extracellular polysaccharide components into the physical state of glass – a strategy known to be exploited by desiccation tolerant plant seeds and water bears (tardigrades). In addition to water, the extracellular space supports molecular self-organization processes. For example, proteins form complexes, while accumulation of minerals templated by organic extracellular matrix may lead to biomineralization of biofilms such as the formation of hardened plaque on the surface of teeth.
How to control physics
One of the exciting future directions of research proposed in the perspective is to understand how physical processes occurring in the extracellular space are regulated at the genetic level of embedded bacteria. The authors argue that the regulatory pathways leading to matrix secretion are relatively well understood. However, how the sequence of matrix production events is orchestrated depending on cell microenvironment, and how it might change in response to external perturbations is an open question. “Yet, very basic physical processes could be key to understanding this complex space-time hierarchical organization from the nanometer scale of individual proteins to whole biofilms at the scale of centimeters” – explains Prof. Vasily Zaburdaev (Friedrich-Alexander-Universität Erlangen-Nürnberg and at the Max-Planck-Zentrum für Physik und Medizin in Erlangen).
This research was supported by the German Science Foundation (DFG) within the Priority Program SPP2389 “Emergent functions of bacterial multicellularity”.
Wissenschaftlicher Ansprechpartner:
Vasily Zaburdaev: Max-Planck-Zentrum für Physik und Medizin / Principal Investigator ›Immunophysics‹
and Friedrich-Alexander-Universität Erlangen-Nürnberg
Chair of Mathematics in the Life Sciences
vasily.zaburdaev@mpzpm.mpg.de
Liraz Chai: Hebrew University of Jerusalem, Israel and Max Planck Queensland Centre, Queensland University of Technology, Brisbane, Australia
liraz.chai@mail.huji.ac.il
Roberto Kolter: Harvard Medical School, Boston, USA
roberto_kolter@hms.harvard.edu
Originalpublikation:
Original publication in Proceedings of the National Academy of Sciences (PNAS)
Liraz Chai, Vasily Zaburdaev, and Roberto Kolter:
“How bacteria actively use passive physics to make biofilms”
DOI: https://doi.org/10.1073/pnas.2403842121
Die semantisch ähnlichsten Pressemitteilungen im idw
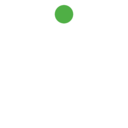